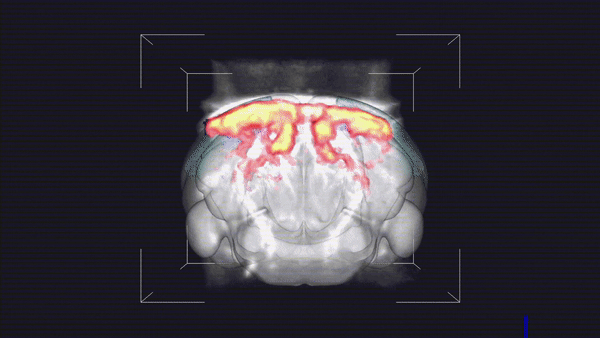
Functional neuroimaging
The high spatial resolution of functional ultrasound allows task-based neuroimaging (also called functional activation mapping) to be used very successfully to investigate brain responses to various stimuli, including visual and auditory inputs.
This was one of the first applications of functional ultrasound, and since 2011 numerous studies have used our technology, as summarized below.
Whisker stimulation
Visual Stimulation
Electrical stimulation
Auditory Stimulation
Pain Stimulation
Physiological stimulation to elicit a brain response is a long-established method of testing brain imaging technology, and in an early study (Macé et al., Nature Methods, 2011) we found that fUS delivered very good sensitivity and spatial resolution for whisker stimulation in the rat.
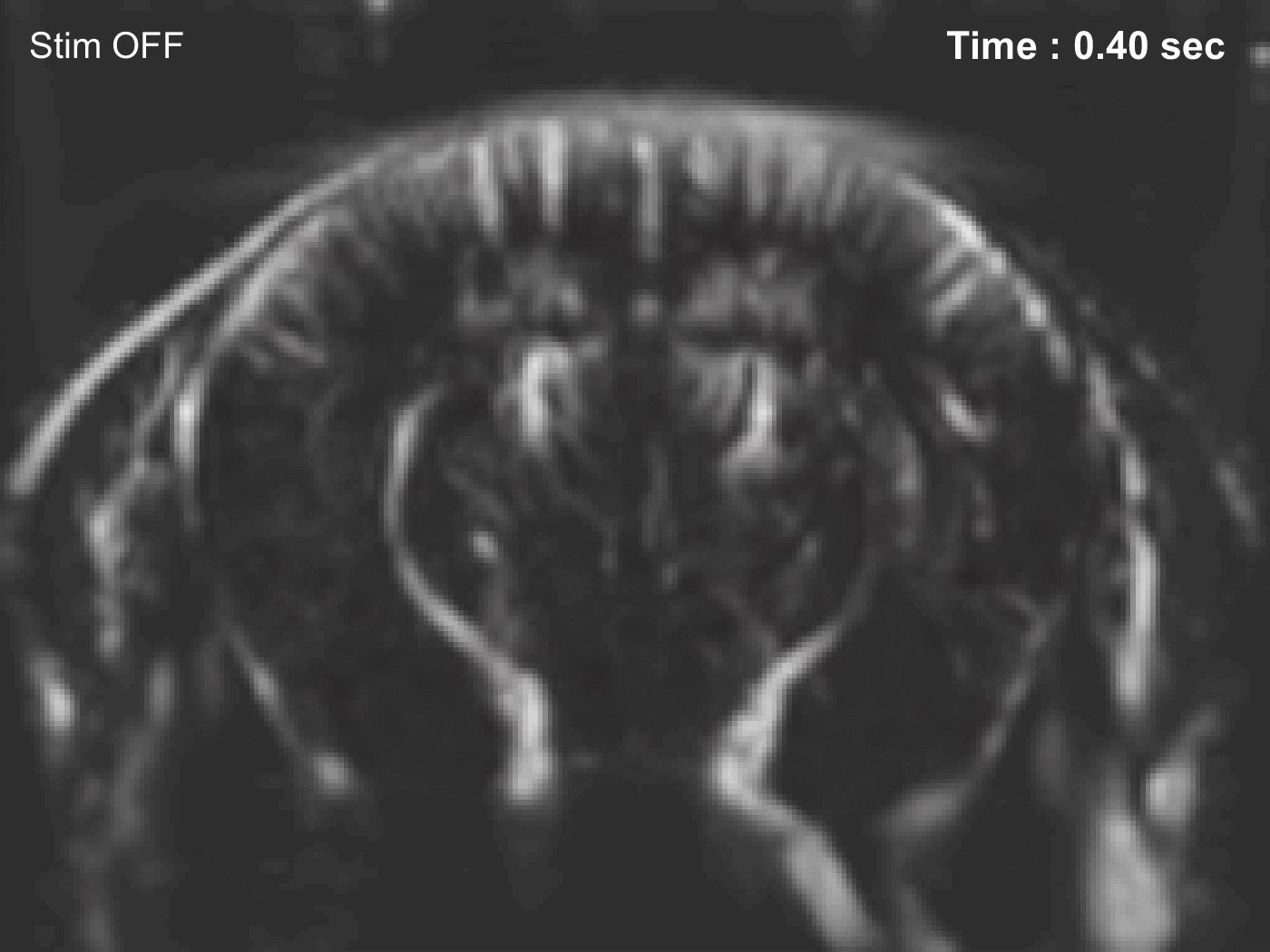
The high spatial resolution of fUS allows the creation of detailed retinotopic maps of animals performing complex visual tasks, as shown in a pioneering study of the rat (see the image below).
Researchers have also shown how fUS can be used to map the regions involved in complex tasks and rule handling in the supplementary eye field. Notably, SEF activation was observed in a single trial, without averaging (see the figure above). fUS neuroimaging is now also being investigated in non-human primates for potential applications in brain–computer interfaces (BCIs).
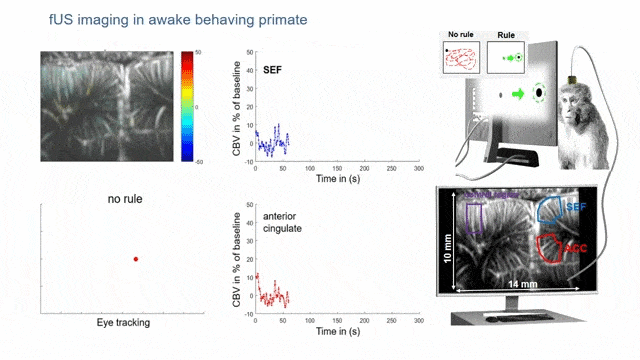
Nature Communications, 2019 (licensed under CC BY 4.0)
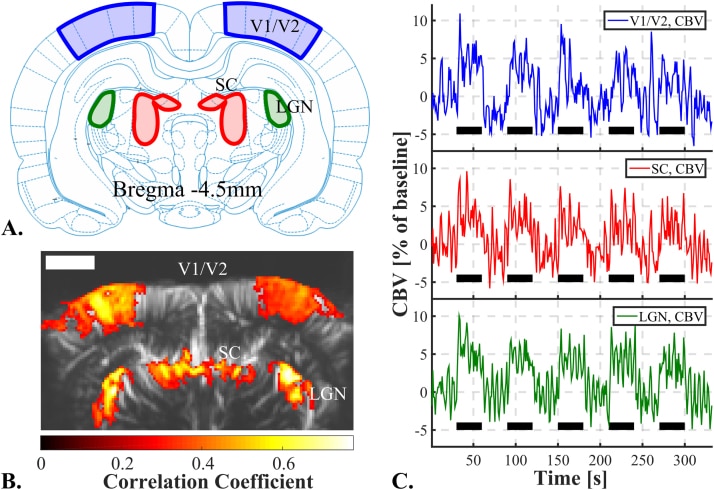
Electrical stimulation is another commonly investigated input, and the study here shows how the responses from fUS are much greater than for fMRI. This means that a single activation is sufficient to achieve good sensitivity, avoiding the need to average multiple experiments in order to increase the signal-to-noise ratio (as with fMRI).
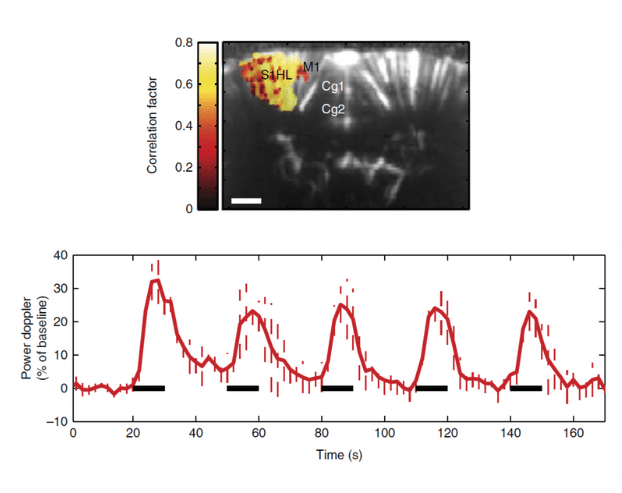
Researchers have also begun to study audition in awake ferrets, an excellent model for hearing research.
Using fUS, they’ve been able to construct highly-detailed sonotopic maps (see the figure). As a result, they were able to obtain independent auditory response curves from voxels just 100 µm apart – an excellent demonstration of the resolution of fUS
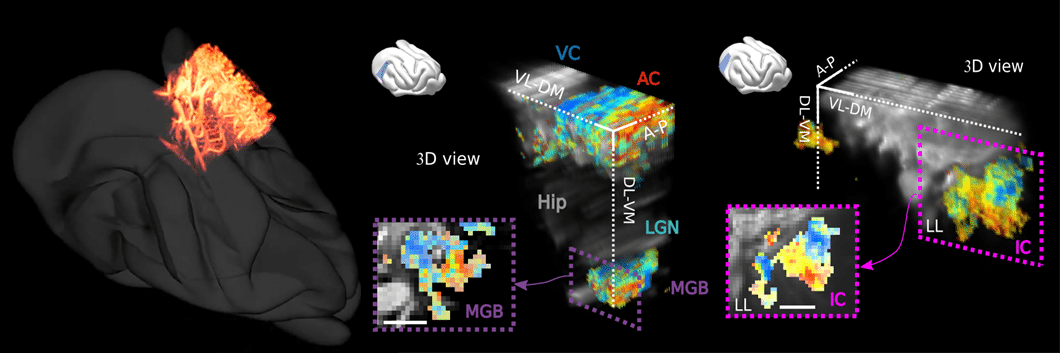
2018 (licensed under CC BY 4.0)
As well as being used to study the brain, fUS can also be used to study the response of the spinal cord to pain induced elsewhere in the body. This is very difficult with fMRI because of the movement due to breathing, but a combination of fUS neuroimaging and ultrasound localization microscopy (ULM) avoids this problem. Responses can therefore be mapped on an impressively small scale (see the figure), with obvious applications to spinal cord damage.
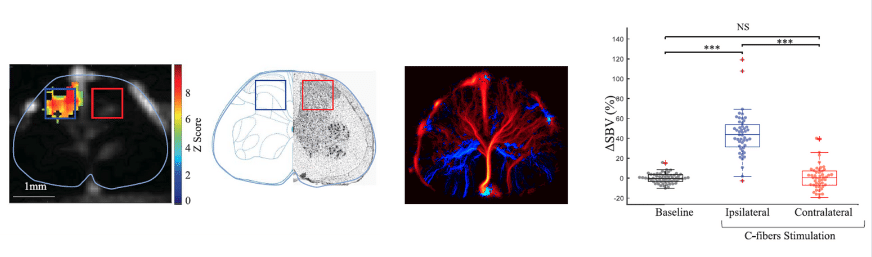
Natural behavior
Finally, pilot studies have shown that fUS probes can be used to measure brain-wide activity in freely-moving rats while exhibiting their natural behavior. This is in contrast to fMRI, which requires the animal to be restrained or anesthetized.
The following two examples – both from the same group – also show that fUS is compatible with EEG recordings, resulting in additional insights into brain activity.
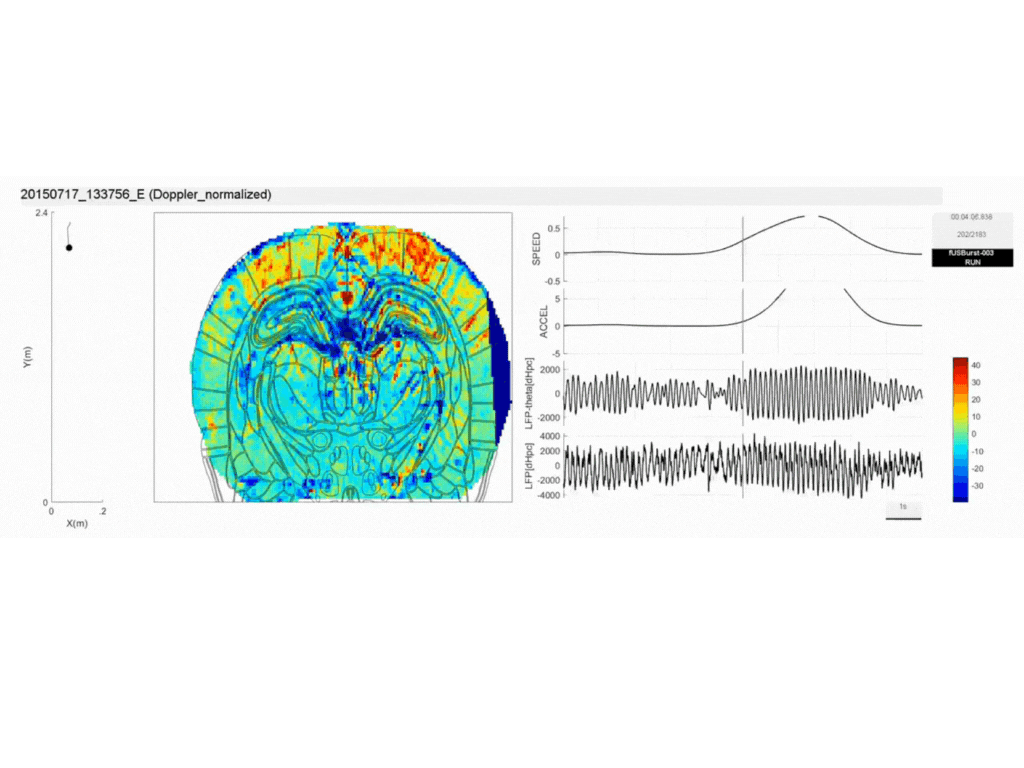
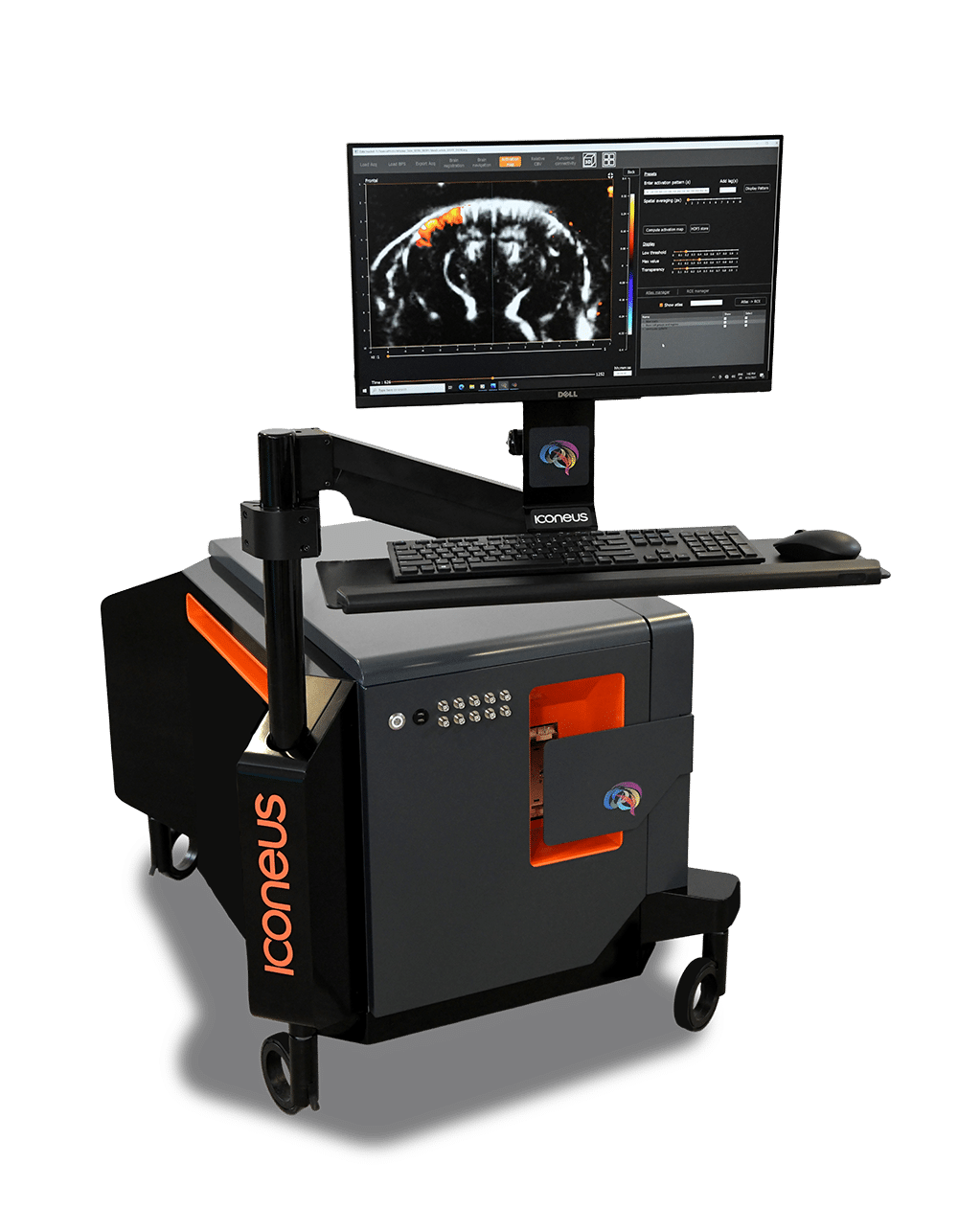
Iconeus One
The only fUS system designed specifically for neuroscientists
Iconeus One is an imaging system with a difference – one with the sensitivity and resolution needed to see what’s happening in the brain at the finest scales. Not only does it work on awake or moving animals, but you can even see what’s happening in real-time.
Contact us
Interested by what you’ve read about functional activation mapping using fUS? Talk to one of our specialists about your application.
Other applications
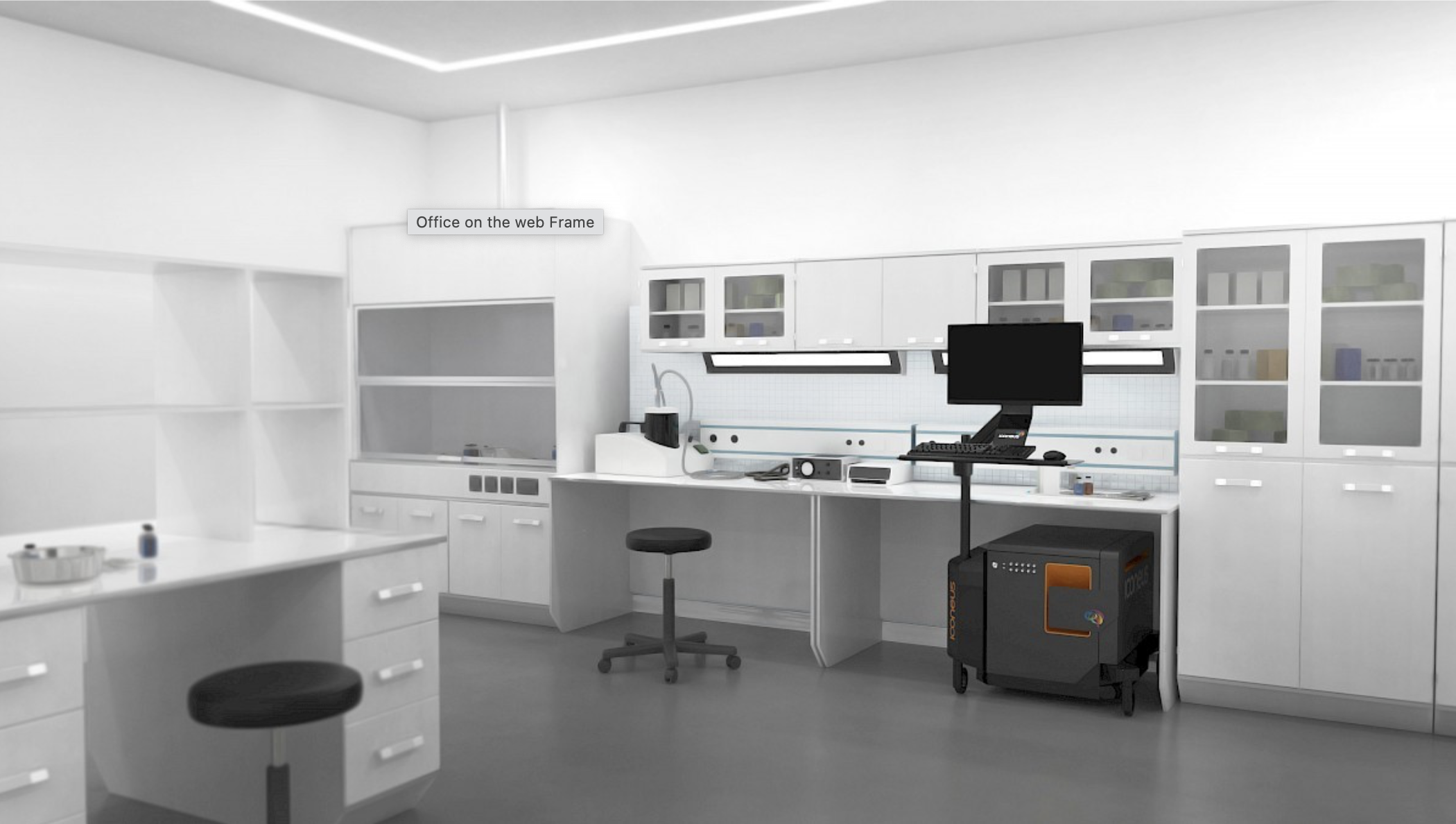